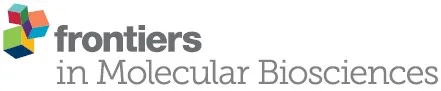
ORIGINAL RESEARCH
published: 20 December 2021
doi: 10.3389/fmolb.2021.747493
OPEN ACCESS
Edited by:
Marc Diederich,
Seoul National University, South Korea
Reviewed by:
Alfonso Pompella,
University of Pisa, Italy,
Alessandro Corti,
University of Pisa, Italy
*Correspondence:
Marija Pljesa-Ercegovac
marija.pljesa-ercegovac@
med.bg.ac.rs
Tatjana Simic
tatjana.simic@med.bg.ac.rs
†These authors have contributed
equally to this work and share first
authorship
Specialty section:
This article was submitted to
Cellular Biochemistry,
a section of the journal
Frontiers in Molecular Biosciences
Received: 26 July 2021
Accepted: 09 November 2021
Published: 20 December 2021
Citation:
Coric V, Milosevic I, Djukic T,
Bukumiric Z, Savic-Radojevic A,
Matic M, Jerotic D, Todorovic N,
Asanin M, Ercegovac M, Ranin J,
Stevanovic G, Pljesa-ErcegovacMand
Simic T (2021) GSTP1 and GSTM3
Variant Alleles Affect Susceptibility and
Severity of COVID-19.
Front. Mol. Biosci. 8:747493.
doi: 10.3389/fmolb.2021.747493
GSTP1 and GSTM3 Variant Alleles Affect Susceptibility and Severity of COVID-19
Vesna Coric1,2†, Ivana Milosevic1,3†, Tatjana Djukic1,2, Zoran Bukumiric1,4, Ana Savic-Radojevic1,2, Marija Matic1,2, Djurdja Jerotic 1,2, Nevena Todorovic3, Milika Asanin1,5, Marko Ercegovac1,6, Jovan Ranin1,3, Goran Stevanovic1,3, Marija Pljesa-Ercegovac1,2* and Tatjana Simic1,2,7*
1Faculty of Medicine, University of Belgrade, Belgrade, Serbia, 2Institute of Medical and Clinical Biochemistry, Belgrade, Serbia, 3Clinic of Infectious and Tropical Diseases, Clinical Centre of Serbia, Belgrade, Serbia, 4Institute of Medical Statistics and Informatics, Belgrade, Serbia, 5Clinic of Neurology, Clinical Centre of Serbia, Belgrade, Serbia, 6Clinic of Cardiology, Clinical Centre of Serbia, Belgrade, Serbia, 7Serbian Academy of Sciences and Arts, Belgrade, Serbia
Based on the premise that oxidative stress plays an important role in severe acute respiratory syndrome coronavirus (SARS-CoV-2) infection, we speculated that variations in the antioxidant activities of different members of the glutathione S-transferase family of enzymes might modulate individual susceptibility towards development of clinical manifestations in COVID-19. The distribution of polymorphisms in cytosolic glutathione S-transferases GSTA1, GSTM1, GSTM3, GSTP1 (rs1695 and rs1138272), and GSTT1 were assessed in 207 COVID-19 patients and 252 matched healthy individuals, emphasizing their individual and cumulative effect in disease
development and severity. GST polymorphisms were determined by appropriate PCR methods. Among six GST polymorphisms analyzed in this study, GSTP1 rs1695 and GSTM3 were found to be associated with COVID-19. Indeed, the data obtained showed that individuals carrying variant GSTP1-Val allele exhibit lower odds of COVID-19
development (p 0.002), contrary to carriers of variant GSTM3-CC genotype which have higher odds for COVID-19 (p 0.024). Moreover, combined GSTP1 (rs1138272 and rs1695) and GSTM3 genotype exhibited cumulative risk regarding both COVID-19
occurrence and COVID-19 severity (p 0.001 and p 0.025, respectively). Further studies are needed to clarify the exact roles of specific glutathione S-transferases once the SARSCoV- 2 infection is initiated in the host cell.
Keywords: oxidative stress, COVID-19, glutathione transferases, GSTP1, GSTM3, polymorphisms
INTRODUCTION
It has been suggested that oxidative stress plays an important role in severe acute respiratory syndrome coronavirus (SARS-CoV-2) infection. Namely, oxidative stress is supposed to mediate various processes in COVID-19, including binding to viral receptor and replication, enhanced cytokine production, inflammation, and cell signaling (Fernandes et al., 2020). Reactive oxygen species (ROS)-induced activation of transcription factors and pro-inflammatory genes triggers immune cells to secrete various cytokines and chemokines, leading to additional ROS generation by immune cells (Chatterjee, 2016). Moreover, redox-sensitive p38 mitogen-activated protein kinases
(MAPK) pathway is shown to be induced in COVID-19, shedding even more light on the importance of disturbed redox homeostasis in SARS-CoV-2 infection (Cheng et al., 2020). It seems that both overproduction of ROS and failure of antioxidant mechanisms affect viral replication and accompanying clinical manifestations of virus-associated disease. Furthermore, tissue damage consequential to ROS overproduction reflects the severity of viral infection (Naumenko et al., 2018). Still, the data on the antioxidant defense system, as an important determinant of redox balance, are scarce. In the view of significant variations in response to SARS-CoV-2 infection, co-morbidities, and suspected role of oxidative stress, it is biologically plausible that inter-individual differences in susceptibility, as well as severity of clinical manifestations in COVID-19 patients, might be affected by their antioxidant genetic profile. Members of the glutathione S-transferase (GST) superfamily, which are known for detoxifying xenobiotics, also exhibit diverse antioxidant and anti-inflammatory roles (Tew and Townsend, 2012; Menon et al., 2017; Pljesa-Ercegovac et al., 2018). Additionally, the involvement of several GSTs in the regulation of signaling pathways, by means of interactions with members of the MAPK signaling pathway (JNK-c-Jun N-terminal kinase, ASK-apoptosis signal-regulating kinase, Akt-protein kinase B) and certain receptors, is well established (Pljesa-Ercegovac et al., 2018). The most important cytosolic GST comprises alpha (GSTA), mi (GSTM), pi (GSTP), and theta (GSTT) classes. Deletion polymorphism in GSTM1 and GSTT1 genes leads to complete lack of enzymatic activity in homozygous carriers of the null genotype. Additionally, GSTM3 and GSTA1 polymorphisms influence gene expression, while two linked GSTP1 single-nucleotide polymorphisms modify substrate specificity. So far, it has been revealed that GSTT1- and GSTM1-null genotypes have differential behavior versus COVID-19 mortality. Namely, individuals with lower frequency of the GSTT1-null genotype exhibit higher COVID-19 mortality (Saadat, 2020a; Abbas et al., 2021) which is important considering the fact that GST genotypes distribution is ethnicity-dependent (Polimanti et al., 2013). Similarly, morbidity and mortality of COVID-19 also correlate with GSTP1-Ile105Val polymorphism in a way that countries with more frequent Val105 allele have higher prevalence and mortality of COVID-19 (Saadat, 2020b).
The potential effect of GSTP1 in COVID-19 should also be analyzed in line with its anti-inflammatory role (Zhou et al., 2018). Surprisingly, the role of established antioxidant GST member, GSTA1, has not been evaluated as yet. Since genetic polymorphisms in the antioxidant defense system are recognized as determinants of risk and prognosis of the major COVID-19 comorbidities, their role in susceptibility to development of COVID-19 clinical manifestations should be clarified. In this line, we aimed to assess the distribution of polymorphisms in genes encoding glutathione transferases alpha (GSTA1), mu (GSTM1, GSTM3), pi (GSTP1 rs1695 and GSTP1 rs1138272), and theta (GSTT1) in COVID-19 patients and matched healthy individuals, emphasizing their individual and cumulative effect in disease development.
MATERIALS AND METHODS
Study Group
The study group comprised 207 COVID-19 patients (120 men and 87 women, with an average age of 52.9 ± 13.9 years) treated at the Institute of Infectious and Tropical Diseases, Clinical Centre of Serbia, between July 2020 and February 2021. Inclusion criteria for participation in the study were as follows: positive SARS-CoV- 2 reverse transcription (RT)-PCR test performed from nasopharyngeal and oropharyngeal swabs according to World Health Organization guidelines and using available RT-PCR protocols, age (≥18 years old), and their willingness to provide written informed consent. Age- and gender-matched control group included 252 individuals (123 men, 129 women; average age 51.5 ± 13.0 years) with confirmed lack of SARS-CoV-2 antibodies (IgM and IgG). The controls were randomly chosen among subjects exposed to the same infection risks as the patient group in order to obtain the groups of homogeneous origin. All participants were Caucasians by ethnicity. The principles of International Conference on Harmonisation (ICH) Good Clinical Practice, the “Declaration of Helsinki,” and national and international ethical guidelines were followed during this study with approval obtained from the Ethics Committee of Clinical Centre of Serbia (566/01 from July 13, 2020 and 608/01 from August 7, 2020). Informed written consent was procured from all recruited subjects.
DNA Isolation and Glutathione Transferases Genotyping
A total DNA was purified from EDTA-anticoagulated peripheral blood obtained from the study participants using PureLink™ Genomic DNA Mini Kit (ThermoFisher Scientific, United States). GSTP1 rs1695, GSTP1 rs1138272, and GSTM3 rs1332018 polymorphisms were determined by real-time PCR on Mastercycler ep realplex (Eppendorf, Germany), using TaqMan Drug Metabolism Genotyping assays (Life Technologies, Applied Biosystems, United States). Assays’ IDs were as follows: C_3237198_20, C_1049615_20, and C_3184522_30, respectively. GSTM1 and GSTT1 deletion polymorphisms were determined by multiplex PCR, using CYP1A1 gene as an internal control. GSTA1 rs3957357 polymorphism was determined by PCRrestriction fragment length polymorphism (PCR-RFLP). Enzymatic digestion of amplified sequence was performed overnight at 37°C using EarI restriction enzyme (Thermo Fisher Scientific, United States). Primers sequences of GSTM1, GSTT1, CYP1A1, and GSTA1 genes and PCR protocols details are given in Table 1. PCR products were separated on 2% agarose gel stained with SYBR® Safe DNA Gel Stain (Invitrogen, United States) and visualized on Chemidoc (Biorad, United States).
Statistical Analysis
Statistical data analysis was performed using IBM SPSS Statistics 22 (SPSS Inc., Chicago, IL, United States). Results were presented as frequency, percent and mean ± SD. Data were analyzed using univariate and multivariate logistic regression for calculating odds ratio (OR) and 95% confidence interval (95%CI) in order to determine the potential association between GST genotypes and odds for the development of COVID-19. Age, gender,
presence of diabetes mellitus type 2, hypertension, obesity, and smoking habits were considered as confounding factors in analysis. All p-values less than 0.05 were considered significant.
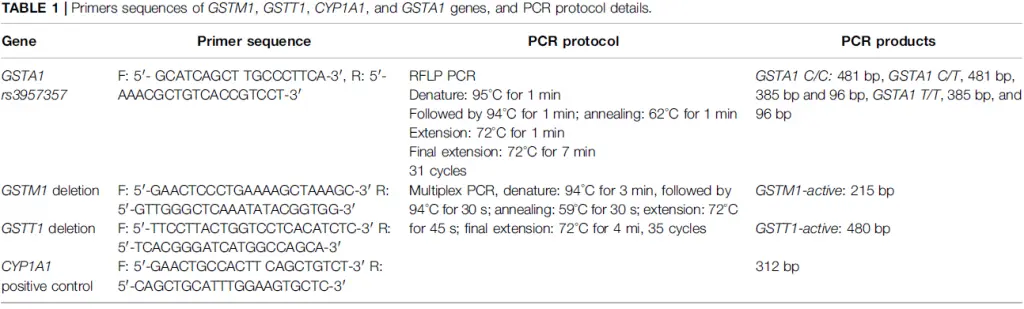
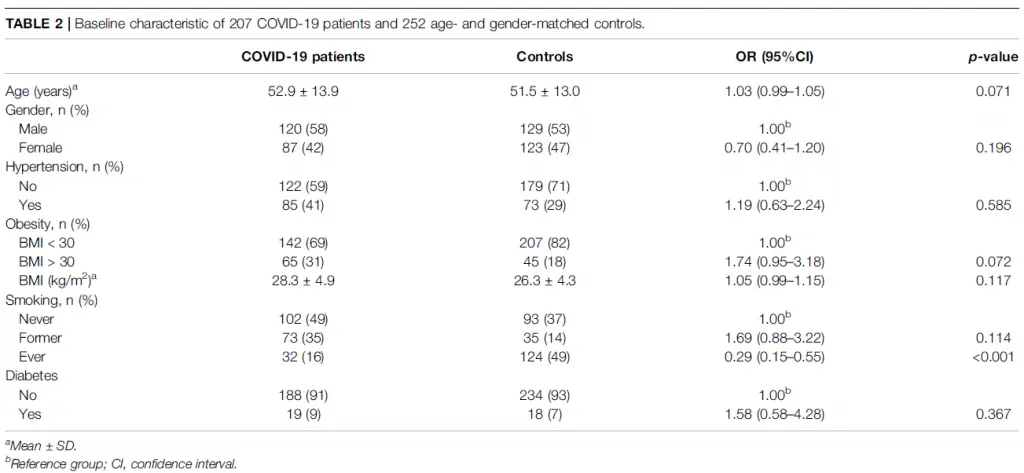
RESULTS
A total of 207 COVID-19 patients and 252 age- and gendermatched controls were included in the study. Baseline characteristics of patients and controls are summarized in Table 2. As presented, most frequent COVID-19 comorbidities, such as diabetes, obesity, and hypertension, were evaluated. No significant difference among groups was found regarding these most frequent comorbidities. However, almost 50% of controls were smokers in comparison with 16% of smokers in the COVID-19 group (p < 0.001). Out of 43% of patients who needed hospitalization, none required mechanical ventilation.
The distribution of specific genotypes among COVID-19 patients and controls is presented in Table 3. Among six investigated GST polymorphisms, significant association between GST genotype and susceptibility for development of COVID-19 clinical manifestations was found for both GSTP1 (rs 1695 and rs1138272) and GSTM3 (rs1332018) polymorphisms. Namely, carriers of heterozygous GSTP1 IleVal rs1695 genotype are less prone to develop COVID-19 (OR 0.66, 95%CI 0.44–0.98, p 0.042). Similarly, individuals with at least one GSTP1* Val allele rs1138272 had significantly lower odds of COVID-19 development (p < 0.05) compared to the carriers of wild-type GSTP1 AlaAla genotype. As for GSTM3 polymorphism, carriers of GSTM3 AC genotype had significantly lower odds of
developing COVID-19 compared to individuals with GSTM3 AA genotype (OR 0.60, 95%CI 0.38–0.96, p 0.033), while homozygous carriers of GSTM3*C allele had 1.7-fold increased COVID-19 odds but with borderline significance (OR 1.71, 95% CI 0.99–2.95, p 0.053). When GST genotypes distribution in COVID-19 patients and controls was assessed including adjustment for age, gender, smoking habit, and comorbidities, comprising hypertension, obesity, and diabetes, significant association remained for GSTP1 (rs1695) and GSTM3 (rs1332018) polymorphisms (Table 4). Namely, we observed that individuals with
GSTP1 IleVal rs 1695 genotype were almost 3-fold less prone for COVID-19 development (OR 0.33, 95%CI 0.17–10.67,
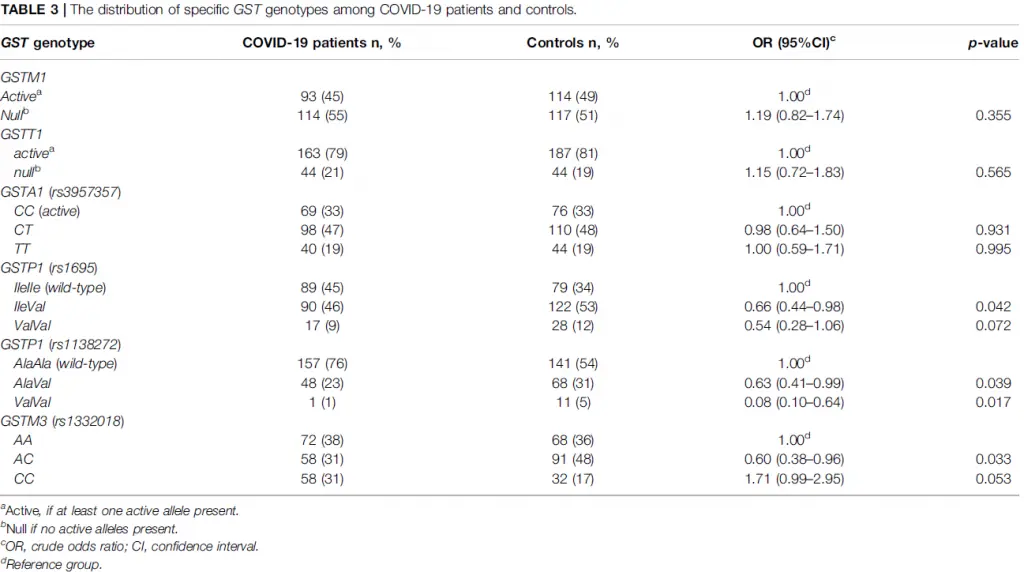
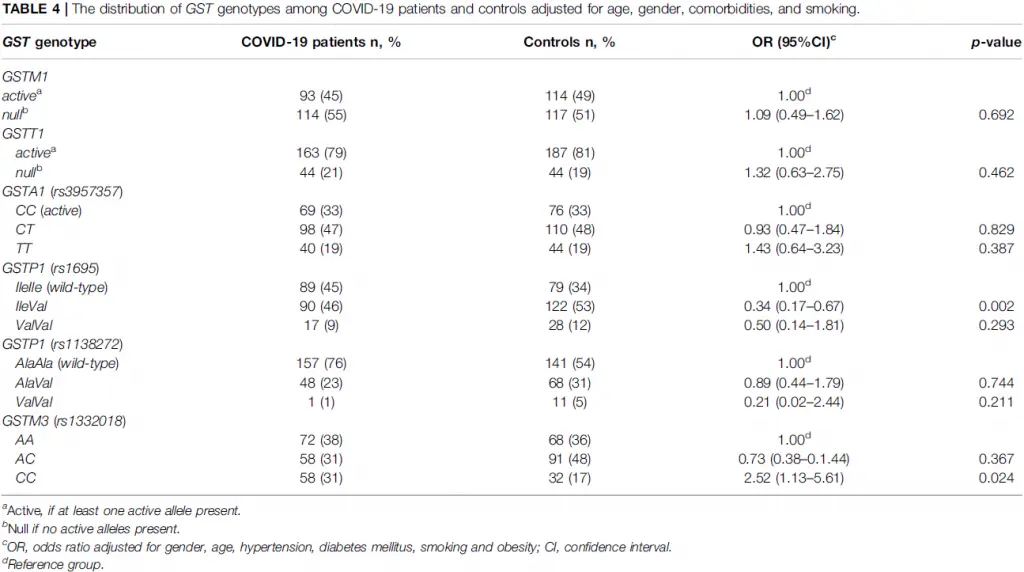
p 0.002) in comparison to the carriers of wild-type GSTP1 IleIle genotype. On the contrary, carriers of GSTM3CC genotype had 2.5-fold higher odds compared to the carriers of GSTM3AA genotype (OR 2.51, 95%CI 1.12–5.61, p 0.024). Finally, the cumulative effect of COVID-19 risk-associated GST genotypes was analyzed in a binary logistic model adjusted for age, gender, comorbidities, and smoking habit. As presented in Table 5, carriers of one, two, or three risk-associated GST genotypes in comparison to the referent category, comprising none of the risk-associated genotypes, exhibited statistically significant increase in odds towards COVID-19. Namely, a trend in OR was observed in COVID-19 patients with one risk-associated genotype, OR 2.76, p 0.012, with two riskassociated genotypes, OR 3.38, p 0.002, while the highest OR was found in patients with three risk-associated genotypes, OR 11.86, p 0.001.
Furthermore, the overall analysis of the number of risk-related GST genotypes was found to be statistically significant (OR 1.88, 95%CI 1.35–2.61, p < 0.001).
Besides, the cumulative effect of GST genotypes was also analyzed in terms of the disease severity. Namely, grouping patients with mild symptoms vs. severe symptoms enabled us to evaluate the prognostic potential of the suggested riskassociated GST genotype combination. The frequency of genotype combination comprising three risk-associated genotypes was the highest in the group of patients with severe COVID-19. Consequently, in these individuals the OR to develop severe form of the disease was 4.7 times higher in comparison with carriers of reference genotype combination (Table 6).
The results on the relation of the assessed GST genotypes with CT scan score, oxygen levels, C-reactive protein (CRP) concentration, and D-dimer and IL-6 levels in the patient’s group are presented in Supplementary Table S1. No significant association was observed for any of the evaluated clinical manifestations of the disease and laboratory parameters. Interestingly, a certain trend was observed regarding CRP values which were the highest in carriers of variant GSTM3-CC
genotype.
DISCUSSION
Based on the premise that oxidative stress plays an important role in SARS-CoV-2 infection, we speculated that variations in antioxidant activities of different members of the GST family of enzymes might modulate individual susceptibility towards the development of clinical manifestations in COVID-19. Among six
GST polymorphisms analyzed in this study, GSTP1 rs1695 and GSTM3 rs1332018 were found to be associated with COVID-19. Indeed, the data obtained showed that individuals carrying variant GSTP1-Val allele exhibit lower odds of COVID-19 development, contrary to the carriers of variant GSTM3-CC genotype who have higher odds for COVID-19. Moreover, combined GSTP1 (rs1138272 and rs1695) and GSTM3 genotype exhibited cumulative risk regarding both OVID-19 occurrence and COVID-19 severity. To our knowledge, this is one the first investigations that addressed the association of common GST olymorphisms and COVID-19. Our results on the association of GSTP1 polymorphisms with risk of COVID-19 seem biologically plausible since GST pi 1 (GSTP1) is highly expressed in lung tissue and might even be considered the predominant GST in the lungs (Terrier et al., 1990; Anttila et al., 1993; Cantlay et al., 1994; Rowe et al., 1997). Although GSTs seem to be ubiquitously expressed in human tissues, the expression of different GST genes may vary significantly between different tissues, giving each organ a unique and complex GST profile (Singh, 2015). Both genetic variants in GSTP1 examined in our study, Ile105Val (rs1695) and Ala114Val (rs1138272), have functional relevance in terms of altered catalytic activity towards a variety of substrates and differences in GSTP1- mediated regulation of redox signaling pathways (Ali-Osman et al., 1997; Di Pietro et al., 2010). Apart from the extensive research on the role of polymorphic GSTP1 expression in various cancers, including lung cancers (Nørskov et al., 2017; Pljesa-Ercegovac et al., 2018; van de Wetering et al., 2021), several studies have also examined the association of these polymorphic variants with susceptibility or outcome in a range of communicable and non-communicable lung diseases (McMillan et al., 2016; van de Wetering et al., 2021). Specifically, in asthma, homozygosity of the GSTP1 Val105 allele is associated with reduced risk of airway hyperresponsiveness and improved lung function (Fryer et al., 2000). Besides, genetic polymorphisms of GSTP1 may be associated with chronic obstructive pulmonary disease (COPD) development suggesting GSTP1-Val allele to be more protective (Ishii et al., 1999). In a large population comprising 66,069 individuals, Norskov et al. showed that GSTP1 Ile105Val genotype was associated with improved lung function, with protection against lung cancer and tobacco-related cancer, aswell as with reduced all-causemortality. The findings weremost pronounced in smokers for all end points, and they even suggested a gene dosage effect (Nørskov et al., 2017). Another lung disease in which GSTP1 inhibition is suggested as a novel therapeutic strategy is lung fibrosis, since GSTP1 is an important participant in protein S-glutathionylation (McMillan et al., 2016). Indeed, the immense group of cellular proteins, the so called “disulfide
proteome” or the “glutathionome” might structurally and functionally be modified by glutathionylation (Lindahl et al., 2011; Pastore and Piemonte, 2012). espite the fact that GSTP1 genetic variability might help our understanding of the susceptibility to COVID-19 disease, the data on the role of GSTP1 in SARS-CoV-2 are scarce. To our knowledge, this is the first case–control study conducted on COVID-19 patients that addressed the role of genetic polymorphisms in GSTP1.Namely, sofar only one study based on the univariable analysis of the World Bank data showed that countries with more frequent Val105 allele have higher prevalence and mortality of COVID-19 (Saadat, 2020b). However, our results on lower susceptibility towards COVID-19 development, obtained in COVID-19 patients, are in line with all the aforementioned studies that support the idea that GSTP1 Val105 plays a protective role in lung function deterioration. One of possible explanations of differential susceptibility among carriers of different GSTP1 alleles may be the variations in regulatory roles of GSTP1. It is noteworthy to mention that, apart from its well-established role in detoxification and antioxidant protection, GSTP1 also exhibits leukotriene synthase activity, thus influencing pulmonary and extrapulmonary manifestations of COVID-19 (Al-Kuraishy et al., 2021). Still, the data on the effect of different GSTP1 genetic variants on LTC4 synthesis are lacking. On the other hand, there are plenty of data regarding the role of GSTP1 in MAPK signaling, with special emphasis on c-Jun-NH2-terminal kinase (JNK) (Board and Menon, 2013). Namely, JNKs are important kinases that are activated in innate immune responses to viral infection and mulate the activity of several significant cytokines, including interleukins (IL- 2, IL-4) (Hemmat et al., 2021). What is more, several SARS-CoV proteins, such as N protein, ORF6 encoded protein, and 3a and 7a proteins were shown to phosphorylate and induce JNK activity in different cell lines potentiating the suggested crucial role of JNK signaling pathway in SARS-CoV infection (Mizutani et al., 2005; Ye et al., 2008; Varshney and Lal, 2011; Fung and Liu, 2017; Hemmat et al., 2021). GSTP1 acts as a negative regulator of JNK kinasedependent apoptotic signaling pathways via GSTP1:JNK1 protein: protein interaction. The complex dissociates in case of increased ROS content, which in turn leads to the association of GSTP1 into oligomers and JNK1 activation. Once activated, JNK1 induces a series of events, starting from the phosphorylation of its substrate, the transcription factor c-Jun, and resulting in apoptosis (Adler et al., 1999; Board and Menon, 2013). It is important to note that the catalytic activity of GSTP1 remains intact even when GSTP1 isengaged in protein:protein interaction, suggesting that the active
site of GSTP1 does not participate in this process (Tew and Townsend, 2012). Based on our findings, it is tempting to speculate that carriers of variant GSTP1 alleles Val105 and Val114, which exhibit better JNK inhibition (Thévenin et al., 2011), are less prone to COVID-19 disease development. Another signaling pathway clearly shown to be disrupted in COVID-19 and involved in hyperinflammatory response is Keap1/Nrf2 [Kelch-like ECH-associated protein 1/nuclear
factor (erythroid-derived 2)-like2] pathway, recognized as a key regulator of cellular redox homeostasis. Namely, specific adaptive cytoprotective response, which includes changes in the Keap1/Nrf2 pathway, is activated when cellular levels of ROS and electrophiles are increased (Chartoumpekis et al., 2015; Basak et al., 2017). Interestingly, Nrf2 regulates GSTP1 gene activation (Vasieva, 2011; Bartolini and Galli, 2016), while at the same time, GSTP1 is capable of forming protein complex with Nrf2, which helps stabilize Nrf2 and its further activity (Bartolini et al., 2015). Still, the functional relevance of GSTP1 polymorphic xpression in terms of Nrf2 stabilization needs to be elucidated. Regarding the role of GST mu class in lung inflammation, GSTM1 polymorphism has been most extensively studied since its lack, or the null genotype, is highly prevalent in the population, and associated with increased risk of inflammatory lung diseases (Wu et al., 2012). Indeed, GSTs, and especially GSTM1, are shown to contribute to enzymatic antioxidant capacity, protecting lungs from cell-derived endogenous and inhaled oxidants (Rahman and MacNee, 2000; Rahman et al., 2006). However, in our case–control study no association between GSTM1 genotype and COVID-19 development was observed. Instead, we found that GSTM3, known to be in linkage disequilibrium with GSTM1, meaning it might influence GSTM1 expression and possess overlapping substrate specificity with GSTM1 (Hayes and Strange, 2000; Hayes et al., 2005), significantly contributes to COVID-19 development. Decades ago, two GSTM3 alleles, GSTM3-A and GSTM3-C, were identified based on the presence of an intronic recognition motif for the Yin Yang
1 (YY1) transcription factor which is known to have a fundamental role in normal biologic processes such as embryogenesis, differentiation, replication, and cellular proliferation (Inskip et al., 1995; Di Pietro et al., 2010). Namely, onemutation of the GSTM3 gene generates a recognition factor for YY1. This might especially be important in the lungs, since evidence suggests that GSTs are important mediators of normal lung growth and their contribution
to the development of lung diseases in adults may already start in utero, continuing through infancy, childhood, and adult life, potentially contributing to so-called early life susceptibility (van de Wetering et al., 2021). Apart from GSTM1, with whom GSTM3 shares an amino acid sequence identity of about 70%, it has around 35% sequence identity with the alpha, pi, and theta GST classes, underlying its role in the detoxification of carcinogenic compounds (Patskovsky et al., 1999). Indeed, GSTM3 has been dominantly associated with various cancers, and changes in its expression are shown to affect the progression of various tumors (Wang et al., 2020), although it has also been associated with COPD and lung disease in children with cystic fibrosis (Flamant et al., 2004; Çalışkan et al., 2015; van de Wetering et al., 2021). The possible explanation for the role of GSTM3 in maintaining redox homeostasis and its established responsiveness to oxidative stress, in both communicable and non-communicable diseases, might be in the fact that the expression of GSTM3 is regulated by the domain- ontaining protein 1 (NSD1), whose H2O2-induced suppression further leads to the reduction of GSTM3 levels through the −63A/C TATA box (Liu et al., 2005; Chu et al., 2014). In this line, it might be speculated that the presence of GSTM3-CC genotype in individuals with SARS-CoV-2 infection might significantly affect antioxidant capacity and consequential oxidative stress-associated mechanisms underlying COVID-19 development. Another rather common deletion polymorphism in genes encoding for human cytosolic GSTs, present in approximately 20% of Caucasians, is GSTT1 gene deletion which results in complete lack
of GSTT1 enzyme activity (Wiencke et al., 1995). It is actually one of the GST polymorphisms so far associatedwith COVID-19.Namely, in his theoretical ecologic study, Saadat suggested that the countries with lower frequency of the GSTT1-null genotype exhibit higher COVID- 19 mortality (Saadat, 2020a). In this line, just recently, Abbas et al. showed that COVID-19 patients with the GSTT1-null genotype have higher mortality rates, while they found no association with susceptibility towards COVID-19 development (Abbas et al., 2021). The results of our case–control study are in agreement with this finding. Last but not least, one single-nucleotide polymorphism in genes encoding common GSTs analyzed in our study is GSTA1 polymorphism. It is represented by three apparently linked SNPs: −567TOG, −69COT, and −52GOA, resulting in differential expression with lower transcriptional activation of the variant GSTA1B (-567G, -69T, -52A) than common GSTA1A allele (−567T, −69C, −52G) (Coles and Kadlubar, 2005). Although the antioxidant role of GSTA1 is well established and certain structural homology betweenGSTA1 andGSTP1 explains whyGSTA1 can also suppress JNK1 signaling in a similar manner as GSTP1 (Romero et al., 2006; Pljesa-Ercegovac et al., 2018), we did not find any association between GSTA1 genotypes and COVID-19 development. Taken together, our results on the association between certain GSTP1 and GSTM3 genetic variants and COVID-19 have shed some light on the involvement of genetic susceptibility in COVID-19 development. Further pointing to the multifacetedrole of GSTP1 as the dominant glutathione transferase class in lungs are the results regarding the role of combined GSTP1 rs1138272 and rs1695) and GSTM3 genotype in both COVID-19 occurrence and severity. However, further studies are needed to clarify the exact roles of specific glutathione transferases once the SARS-CoV-2 infection is initiated in the host cell. Taking into consideration the role of different GSTs in the regulation of redox signaling pathways, our results might even contribute to better identification of potential targets for novel drugs that might aid patient treatment in this pandemic.
DATA AVAILABILITY STATEMENT
The datasets presented in this study can be found in online repositories. The names of the repository/repositories and accession number(s) can be found at: https://redcap.med.bg.ac. rs/, AntioxIdentification.
ETHICS STATEMENT
The studies involving human participants were reviewed and approved by the Ethics Committee of Clinical Centre of Serbia (566/01 from July 13, 2020, and 608/01 from August 7, 2020). The patients/participants provided their written informed consent to participate in this study.
AUTHOR CONTRIBUTIONS
TS, MP-E, and GS designed the study. IM, GS, JR, NT, MA, and ME recruited and screened the participants. VC, TD, and DJ performed the genetic analyses. ZB performed the statistical analysis. TS, MP-E, GS, AS-R, JR, TD, MM, VC, IM, MA, and ME performed the literature search and wrote the article. VC and IM ontributed equally as first authors of the article. TS and MP-E are co-corresponding authors. All authors contributed to the article and approved the submitted version.
FUNDING
This work was supported by the Special Research Program COVID-19, Project No7546803 AntioxIdentification, Science Fund of the Republic of Serbia as well as Ministry of Education, Science and Technological Development of the Republic of Serbia (Grant No. 200110).
ACKNOWLEDGMENTS
We wish to thank Sanja Sekulic for technical support.
SUPPLEMENTARY MATERIAL
The Supplementary Material for this article can be found online at: https://www.frontiersin.org/articles/10.3389/fmolb.2021.747493/full#supplementary-material
REFERENCES
Abbas, M., Verma, S., Verma, S., Siddiqui, S., Khan, F. H., Raza, S. T., et al. (2021).
Association of GSTM1 and GSTT1 Gene Polymorphisms with COVID-19
Susceptibility and its Outcome. J. Med. Virol. 93 (9), 5446–5451. doi:10.1002/
jmv.27076
Adler, V., Yin, Z., Fuchs, S. Y., Benezra, M., Rosario, L., Tew, K. D., et al. (1999).
Regulation of JNK Signaling by GSTp. EMBO J. 18 (5), 1321–1334. doi:10.1093/
emboj/18.5.1321
Al-Kuraishy, H. M., Al-Gareeb, A. I., Almulaiky, Y. Q., Cruz-Martins, N., and El-
Saber Batiha, G. (2021). Role of Leukotriene Pathway and Montelukast in
Pulmonary and Extrapulmonary Manifestations of Covid-19: The Enigmatic
Entity. Eur. J. Pharmacol. 904, 174196. doi:10.1016/j.ejphar.2021.174196
Ali-Osman, F., Akande, O., Antoun, G., Mao, J.-X., and Buolamwini, J. (1997).
Molecular Cloning, Characterization, and Expression in Escherichia C of Full-
Length cDNAs of Three Human Glutathione S-Transferase Pi Gene Variants.
J. Biol. Chem. 272 (15), 10004–10012. doi:10.1074/jbc.272.15.10004
Anttila, S., Hirvonen, A., Vainio, H., Husgafvel-Pursiainen, K., Hayes, J. D., and
Ketterer, B. (1993). Immunohistochemical Localization of Glutathione
S-Transferases in Human Lung. Cancer Res. 53 (23), 5643–5648.
Bartolini, D., Commodi, J., Piroddi, M., Incipini, L., Sancineto, L., Santi, C., et al.
(2015). Glutathione S-Transferase Pi Expression Regulates the Nrf2-Dependent
Response to Hormetic Diselenides. Free Radic. Biol. Med. 88, 466–480.
doi:10.1016/j.freeradbiomed.2015.06.039
Bartolini, D., and Galli, F. (2016). The Functional Interactome of GSTP: A
Regulatory Biomolecular Network at the Interface with the Nrf2 Adaption
Response to Oxidative Stress. J. Chromatogr. B 1019, 29–44. doi:10.1016/
j.jchromb.2016.02.002
Basak, P., Sadhukhan, P., Sarkar, P., and Sil, P. C. (2017). Perspectives of the Nrf-2
Signaling Pathway in Cancer Progression and Therapy. Toxicol. Rep. 4 (4),
306–318. doi:10.1016/j.toxrep.2017.06.002
Board, P. G., and Menon, D. (2013). Glutathione Transferases, Regulators of
Cellular Metabolism and Physiology. Biochim. Biophys. Acta (Bba) – Gen.
Subjects 1830 (5), 3267–3288. doi:10.1016/j.bbagen.2012.11.019
Çalışkan, M., Baker, S. W., Gilad, Y., and Ober, C. (2015). Host Genetic Variation
Influences Gene Expression Response to Rhinovirus Infection. Plos Genet. 11
(4), e1005111. doi:10.1371/journal.pgen.1005111
Cantlay, A. M., Smith, C. A., Wallace, W. A., Yap, P. L., Lamb, D., and Harrison, D.
J. (1994). Heterogeneous Expression and Polymorphic Genotype of
Glutathione S-Transferases in Human Lung. Thorax 49 (10), 1010–1014.
doi:10.1136/thx.49.10.1010
Chartoumpekis, D. V., Wakabayashi, N., and Kensler, T. W. (2015). Keap1/Nrf2
Pathway in the Frontiers of Cancer and Non-Cancer Cell Metabolism. Biochem.
Soc. Trans. 43 (4), 639–644. doi:10.1042/bst20150049
Chatterjee, S. (2016). “Oxidative Stress, Inflammation, and Disease,” in Oxidative
Stress and Biomaterials (Amsterdam: Elsevier), 35–58. doi:10.1016/b978-0-12-
803269-5.00002-4
Cheng, Y., Sun, F., Wang, L., Gao, M., Xie, Y., Sun, Y., et al. (2020). Virus-Induced
P38 MAPK Activation Facilitates Viral Infection. Theranostics 10 (26),
12223–12240. doi:10.7150/thno.50992
Chu, G., Li, Y., Dong, X., Liu, J., and Zhao, Y. (2014). Role of NSD1 in H2O2-
Induced GSTM3 Suppression. Cell Signal. 26 (12), 2757–2764. doi:10.1016/
j.cellsig.2014.08.026
Coles, B. F., and Kadlubar, F. F. (2005). Human Alpha Class Glutathione STransferases:
Genetic Polymorphism, Expression, and Susceptibility to Disease.
Methods Enzymol. 401, 9–42. doi:10.1016/s0076-6879(05)01002-5
Di Pietro, G., Magno, L. A. V., and Rios-Santos, F. (2010). Glutathione
S-Transferases: An Overview in Cancer Research. Expert Opin. Drug Metab.
Toxicol. 6 (2), 153–170. doi:10.1517/17425250903427980
Fernandes, I. G., De Brito, C. A., Dos Reis, V. M. S., Sato, M. N., and Pereira, N. Z.
(2020). SARS-CoV-2 and Other Respiratory Viruses: What Does Oxidative
Stress Have to Do with it? Oxid Med. Cel Longev 2020, 8844280. doi:10.1155/
2020/8844280
Flamant, C., Henrion-Caude, A., Boëlle, P.-Y., Brëmont, F. o., Brouard, J., Delaisi,
B., et al. (2004). Glutathione-S-transferase M1, M3, P1 and T1 Polymorphisms
and Severity of Lung Disease in Children with Cystic Fibrosis.
Pharmacogenetics 14 (5), 295–301. doi:10.1097/00008571-200405000-00004
Fryer, A. A., Bianco, A., Hepple, M., Jones, P.W., Strange, R. C., and Spiteri, M. A.
(2000). Polymorphism at the GlutathioneS-Transferase GSTP1 Locus. Am.
J. Respir. Crit. Care Med. 161 (5), 1437–1442. doi:10.1164/
ajrccm.161.5.9903006
Fung, T. S., and Liu, D. X. (2017). Activation of the C-Jun NH2-Terminal Kinase
Pathway by Coronavirus Infectious Bronchitis Virus Promotes Apoptosis
Independently of C-Jun. Cell Death Dis 8 (12), 3215. doi:10.1038/s41419-
017-0053-0
Hayes, J. D., Flanagan, J. U., and Jowsey, I. R. (2005). Glutathione Transferases.
Annu. Rev. Pharmacol. Toxicol. 45, 51–88. doi:10.1146/
annurev.pharmtox.45.120403.095857
Hayes, J. D., and Strange, R. C. (2000). Glutathione S-Transferase Polymorphisms
and Their Biological Consequences. Pharmacology 61 (3), 154–166.
doi:10.1159/000028396
Hemmat, N., Asadzadeh, Z., Ahangar, N. K., Alemohammad, H., Najafzadeh, B.,
Derakhshani, A., et al. (2021). The Roles of Signaling Pathways in SARS-CoV-2
Infection; Lessons Learned from SARS-CoV and MERS-CoV. Arch. Virol. 166
(3), 675–696. doi:10.1007/s00705-021-04958-7
Inskip, A., Elexperu-Camiruaga, J., Buxton, N., Dias, P. S., MacIntosh, J., Campbell,
D., et al. (1995). Identification of Polymorphism at the Glutathione
S-Transferase, GSTM3 Locus: Evidence for Linkage with GSTM1*A.
Biochem. J. 312 (3), 713–716. doi:10.1042/bj3120713
Ishii, T., Matsuse, T., Teramoto, S., Matsui, H., Miyao, M., Hosoi, T., et al. (1999).
Glutathione S-Transferase P1 (GSTP1) Polymorphism in Patients with Chronic
Obstructive Pulmonary Disease. Thorax 54 (8), 693–696. doi:10.1136/
thx.54.8.693
Lindahl, M., Mata-Cabana, A., and Kieselbach, T. (2011). The Disulfide
Proteome and Other Reactive Cysteine Proteomes: Analysis and
Functional Significance. Antioxid. Redox Signaling 14 (12), 2581–2642.
doi:10.1089/ars.2010.3551
Liu, X., Campbell, M. R., Pittman, G. S., Faulkner, E. C.,Watson,M. A., and Bell, D.
A. (2005). Expression-Based Discovery of Variation in the Human Glutathione
S-Transferase M3 Promoter and Functional Analysis in a Glioma Cell Line
Using Allele-specific Chromatin Immunoprecipitation. Cancer Res. 65 (1),
99–104. https://cancerres.aacrjournals.org/content/canres/65/1/99.full.pdf.
McMillan, D. H., van der Velden, J. L., Lahue, K. G., Qian, X., Schneider, R. W.,
Iberg, M. S., et al. (2016). Attenuation of Lung Fibrosis in Mice with a Clinically
Relevant Inhibitor of Glutathione-S-Transferase π. JCI insight 1 (8), e85717.
doi:10.1172/jci.insight.85717
Menon, D., Innes, A., Oakley, A. J., Dahlstrom, J. E., Jensen, L. M., Brüstle, A.,
et al. (2017). GSTO1-1 Plays a Pro-Inflammatory Role in Models of
Inflammation, Colitis and Obesity. Sci. Rep. 7 (1), 17832. doi:10.1038/
s41598-017-17861-6
Mizutani, T., Fukushi, S., Saijo, M., Kurane, I., and Morikawa, S. (2005). JNK and
PI3k/Akt Signaling Pathways Are Required for Establishing Persistent SARSCoV
Infection in Vero E6 Cells. Biochim. Biophys. Acta 1741 (1–2), 4–10.
doi:10.1016/j.bbadis.2005.04.004
Naumenko, V., Turk, M., Jenne, C. N., and Kim, S.-J. (2018). Neutrophils in
Viral Infection. Cell Tissue Res 371 (3), 505–516. doi:10.1007/s00441-
017-2763-0
Nørskov, M. S., Dahl, M., and Tybjærg-Hansen, A. (2017). Genetic Variation in
GSTP1, Lung Function, Risk of Lung Cancer, and Mortality. J. Thorac. Oncol.
12 (11), 1664–1672. doi:10.1016/j.jtho.2017.07.008
Pastore, A., and Piemonte, F. (2012). S-Glutathionylation Signaling in Cell Biology:
Progress and Prospects. Eur. J. Pharm. Sci. 46 (5), 279–292. doi:10.1016/
j.ejps.2012.03.010
Patskovsky, Y. V., Huang, M.-Q., Takayama, T., Listowsky, I., and Pearson, W. R.
(1999). Distinctive Structure of the HumanGSTM3Gene-Inverted Orientation
Relative to the Mu Class Glutathione Transferase Gene Cluster. Arch. Biochem.
Biophys. 361 (1), 85–93. doi:10.1006/abbi.1998.0964
Pljesa-Ercegovac, M., Savic-Radojevic, A., Matic, M., Coric, V., Djukic, T., Radic,
T., et al. (2018). Glutathione Transferases: Potential Targets to Overcome
Chemoresistance in Solid Tumors. Int. J. Mol. Sci. 19 (12), 3785. doi:10.3390/
ijms19123785
Polimanti, R., Carboni, C., Baesso, I., Piacentini, S., Iorio, A., De Stefano, G. F., et al.
(2013). Genetic Variability of Glutathione S-Transferase Enzymes in Human
Populations: Functional Inter-Ethnic Differences in Detoxification Systems.
Gene 512 (1), 102–107. doi:10.1016/j.gene.2012.09.113
Rahman, I., Biswas, S. K., and Kode, A. (2006). Oxidant and Antioxidant Balance in
the Airways and Airway Diseases. Eur. J. Pharmacol. 533 (1–3), 222–239.
doi:10.1016/j.ejphar.2005.12.087
Rahman, I., and MacNee, W. (2000). Oxidative Stress and Regulation of
Glutathione in Lung Inflammation. Eur. Respir. J. 16 (3), 534–554.
doi:10.1034/j.1399-3003.2000.016003534.x
Romero, L., Andrews, K., Ng, L., O’Rourke, K., Maslen, A., and Kirby, G. (2006).
Human GSTA1-1 Reduces C-Jun N-Terminal Kinase Signalling and Apoptosis
in Caco-2 Cells. Biochem. J. 400 (1), 135–141. doi:10.1042/bj20060110
Rowe, J. D., Nieves, E., and Listowsky, I. (1997). Subunit Diversity and Tissue
Distribution of Human Glutathione S-Transferases: Interpretations Based on
Electrospray Ionization-MS and Peptide Sequence-Specific Antisera. Biochem.
J. 325 (2), 481–486. doi:10.1042/bj3250481
Saadat, M. (2020). TheMorbidity and Mortality of COVID-19 Are Correlated with
the Ile105Val Glutathione S-Transferase P1 Polymorphism. Egypt. J. Med.
Hum. Genet. 21 (1), 1–5. doi:10.1186/s43042-020-00094-0
Saadat, M. (2020). An Evidence for Correlation between the Glutathione
S-Transferase T1 (GSTT1) Polymorphism and Outcome of COVID-19.
Clinica Chim. Acta 508, 213–216. doi:10.1016/j.cca.2020.05.041
Singh, S. (2015). Cytoprotective and Regulatory Functions of Glutathione
S-Transferases in Cancer Cell Proliferation and Cell Death. Cancer
Chemother. Pharmacol. 75 (1), 1–15. doi:10.1007/s00280-014-2566-x
Terrier, P., Townsend, A. J., Coindre, J. M., Triche, T. J., and Cowan, K. H. (1990).
An Immunohistochemical Study of Pi Class Glutathione S-Transferase
Expression in normal Human Tissue. Am. J. Pathol. 137 (4), 845–853.
Tew, K. D., and Townsend, D. M. (2012). Glutathione-s-Transferases as
Determinants of Cell Survival and Death. Antioxid. Redox Signaling 17 (12),
1728–1737. doi:10.1089/ars.2012.4640
Thévenin, A. F., Zony, C. L., Bahnson, B. J., and Colman, R. F. (2011). GSTpi
Modulates JNK Activity through a Direct Interaction with JNK Substrate,
ATF2. Protein Sci. 20 (5), 834–848. doi:10.1002/pro.609
van deWetering, C., Elko, E., Berg,M., Schiffers, C.H. J., Stylianidis, V., van den
Berge, M., et al. (2021). Glutathione S-Transferases and Their Implications in
the Lung Diseases Asthma and Chronic Obstructive Pulmonary Disease:
Early Life Susceptibility? Redox Biol. 43, 101995. doi:10.1016/
j.redox.2021.101995
Varshney, B., and Lal, S. K. (2011). SARS-CoV Accessory Protein 3b Induces AP-1
Transcriptional Activity through Activation of JNK and ERK Pathways.
Biochemistry 50 (24), 5419–5425. doi:10.1021/bi200303r
Vasieva, O. (2011). The Many Faces of Glutathione Transferase Pi. Curr. Mol. Med.
11 (2), 129–139. doi:10.2174/156652411794859278
Wang, S., Yang, J., You, L., Dai, M., and Zhao, Y. (2020). GSTM3 Function and
Polymorphism in Cancer: Emerging but Promising. Cancer Manag. Res. 12,
10377–10388. doi:10.2147/cmar.s272467
Wiencke, J. K., Pemble, S., Ketterer, B., and Kelsey, K. T. (1995). Gene Deletion of
Glutathione S-Transferase Theta: Correlation with Induced Genetic Damage
and Potential Role in Endogenous Mutagenesis. Cancer Epidemiol. Biomarkers
Prev. 4 (3), 253–259.
Wu, W., Peden, D., and Diaz-Sanchez, D. (2012). Role of GSTM1 in Resistance to
Lung Inflammation. Free Radic. Biol. Med. 53 (4), 721–729. doi:10.1016/
j.freeradbiomed.2012.05.037
Ye, Z., Wong, C. K., Li, P., and Xie, Y. (2008). A SARS-CoV Protein, ORF-6,
Induces Caspase-3 Mediated, ER Stress and JNK-dependent Apoptosis.
Biochim. Biophys. Acta (Bba) – Gen. Subjects 1780 (12), 1383–1387.
doi:10.1016/j.bbagen.2008.07.009
Zhou, Y., Cao, X., Yang, Y., Wang, J., Yang, W., Ben, P., et al. (2018). Glutathione
S-Transferase Pi Prevents Sepsis-Related High Mobility Group Box-1 Protein
Translocation and Release. Front. Immunol. 9, 268. doi:10.3389/
fimmu.2018.00268
Conflict of Interest: The authors declare that the research was onducted in the absence of any commercial or financial elationships that could be construed as a potential conflict of interest.
Publisher’s Note: All claims expressed in this article are solely those of the authors and do not necessarily represent those of their affiliated organizations or those of the publisher, the editors, and the reviewers. Any product that may be evaluated in this article, or claim that may be made by its manufacturer, is not guaranteed or
endorsed by the publisher.
Copyright © 2021 Coric, Milosevic, Djukic, Bukumiric, Savic- adojevic, Matic, Jerotic, Todorovic, Asanin, Ercegovac, Ranin, Stevanovic, Pljesa-Ercegovac and Simic. This is an open-access article distributed under the terms of the Creative Commons Attribution License (CC BY). The use, distribution or reproduction in
other forums is permitted, provided the original author(s) and the copyright owner(s) are credited and that the original publication in this journal is cited, in accordance with accepted academic practice. No use, distribution or reproduction is permitted which does not comply with these terms.